What Is a Fiber Laser?
A fiber laser can be defined as a solid-state laser that makes use of optical fibers as its active gain medium. A fiber made of glass silicate or phosphate absorbs unprocessed light from the pump laser diodes and converts it into a concentrated beam of a certain wavelength. The optical fiber is doped (incorporating a rare-earth element into the fiber) to make this possible. Different doping substances produce laser beams of differing wavelengths. Some popular doping elements include: dysprosium (2600-3400 nm), holmium (2025-2200 nm), and thulium (1900-2500 nm).
Fiber lasers are among the more energy-efficient laser options available, being up to 50% more energy efficient than CO2 lasers. There are several classification systems based on the: laser source, mode of operation, laser power, and mode (the size of the optical fiber's core, through which light flows).
Given the vast range of possible wavelengths, fiber lasers are ideal for a number of applications including laser cutting, cleaning, texturing, engraving, drilling, marking, and welding. This also makes it possible for fiber lasers to be used in many industries, including materials processing and manufacturing, spectroscopy, electricity generation, transportation, medical applications, transportation, telecommunications, and military hardware.
Although the fiber laser was invented in 1961, the technology didn’t mature enough for commercial use until the 1990s. Most applications demand at least 20 W worth of laser power, whereas early fiber lasers could only output a few dozen milliwatts. Additionally, there was no way to produce high-quality pump light since early laser diodes were simply not efficient enough.
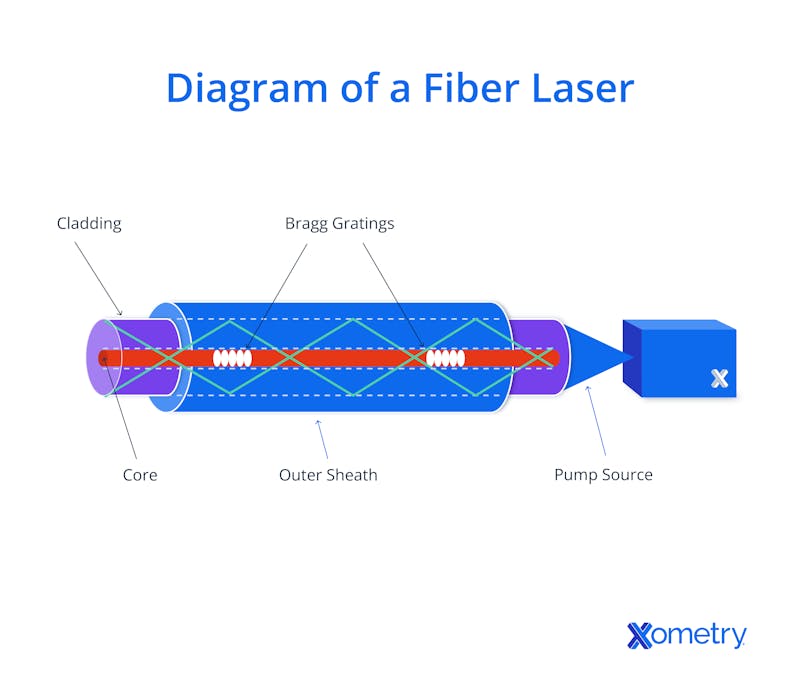
Advantages and Disadvantages of Using a Fiber Laser
In comparison to conventional laser heat sources, the fiber laser has a number of advantages as listed below:
- A fiber laser creates a beam inside the fiber, eliminating the need for a separate optical medium for beam delivery. Therefore, it is incredibly stable and simple to maintain.
- Fiber lasers offer extremely high optical gain. They’re capable of generating a kilowatt of continuous output power.
- Fiber lasers have a 30-50% power conversion rate compared to CO2 lasers' 10-15%, giving them a clear advantage in terms of energy efficiency.
- Fiber lasers are highly accurate even in complex designs because of the focused narrow beams. Since cutting laser devices do not physically contact the workpiece material and only a limited area is affected by heat, the cut remains clean and free of burrs, rough edges, or thermal deformation.
- The fiber's tiny core produces the beam. As a result, it is possible to create a high-quality, straight optical beam with less diffraction than with other methods.
- A fiber laser setup doesn't require routine maintenance like mirror replacement or adjustment. In contrast to CO2 lasers, fiber lasers have no blower or moving parts.
- Fiber lasers use less power and require less maintenance than others, which results in lower operating costs.
Fiber lasers have some disadvantages; these are listed below:
- A fiber laser cutter typically has more expensive components.
- The delivery fiber cannot be separated from the source, making replacement a challenge.
Fiber Laser Applications
Fiber lasers are useful in a variety of applications due to the many power levels they can produce. Among them are:
- Laser Marking: 1064 nm emission wavelength ytterbium-doped fiber lasers are considered ideal for laser marking applications. These lasers may leave sharp, durable imprints on plastic and metal surfaces. They can be customized to accommodate quick production cycles and can be manual or automated. Fiber laser equipment can be used to anneal, etch, and engrave as well.
- Laser Cleaning: Laser cleaning — the process of removing paint, oxide, and rust from metal surfaces — works best using fiber lasers. The procedure can be automated and customized for various manufacturing line conditions.
- Laser Welding: Fiber lasers play a significant role in the welding industry. In comparison to conventional methods, laser welding offers faster speeds, more precision, less distortion, higher quality, and greater efficiency.
- Laser Cutting: Fiber lasers have remarkably effective edge quality and can handle difficult cuts. They are ideal for items with tight tolerances. Due to the extensive list of advantages, fabricators have increasingly begun to favor fiber.
How The Power of a Fiber Laser Scales
Fiber lasers' ability to scale in power is constrained by Brillouin and Raman scattering as well as the short length of the lasers themselves. Many components, including amplifiers, switches, and logic elements, require nonlinear fiber configurations.
There are two classes of nonlinear effects in optical fibers. The first one is brought on by the Kerr effect, or the intensity dependence of the medium's refractive index. This phenomenon manifests as one of three effects, depending on the type of input signal: cross-phase modulation (CPM), self-phase modulation (SPM), or four-wave mixing (FWM).
The second nonlinear effect occurs when the optical field transfers some of its energy to the nonlinear medium via inelastic scattering. Such inelastic scattering can result in phenomena like stimulated Brillouin scattering (SBS) and stimulated Raman scattering (SRS).
Any form of stimulated scattering action can potentially be a source of gain for the fiber. In both processes, if the incident power rises above a specific threshold, the intensity of dispersed light increases exponentially. Because of the comparatively large frequency shift and the wider gain bandwidth, Raman amplification is more beneficial. The main distinction between them is that in Brillouin, the optical wave interacts with low-frequency acoustic phonons, whereas in Raman, the directed optical wave interacts with high-frequency optical phonons. Another key distinction is that SRS can happen in both directions while SBS only happens in the backward direction in optical fibers.
Alternatives to Fiber Lasers
Some alternative cutting technologies to fiber lasers include:
- Gas/CO2 Lasers: A CO2 laser's wavelength is 10.6 mm. Compared to a fiber laser with the same power output, a CO2 laser has more energy to cut through thicker materials and can produce a smoother finish. Materials that can be cut with CO2 lasers include acrylic, leather, some plastics and foams, glass, paper-based materials, and wood.
- Crystal Laser Cutters: Crystal laser cutters operate at shorter wavelengths compared to CO2 lasers, meaning they have higher intensities, allowing them to cut through thicker and stronger materials. However, because of their high power, their parts deteriorate quickly. Crystal lasers are most often used to cut metals, ceramics, and plastics.
Materials that a Fiber Laser Can Cut
Fiber lasers can be used to cut the following materials:
- Metals such as: carbon steel, copper, brass, stainless steel, titanium, and aluminum.
- Plastic such as: acrylic, polyoxymethylene, and lucite.
- Graphite
On the other hand, fiber laser cutters are not suitable for: glass fiber, leather, ceramics, polycarbonate, ABS, HDPE, polystyrene, and polypropylene foam.
The Main Types of Fiber Lasers
The main types of fiber lasers are classified depending on the laser source (thulium-doped, erbium-doped, or ytterbium-doped), mode, mode of operation (multi-mode or single-mode), and laser power.
How To Classify Fiber Laser Types
The following can be used to classify fiber lasers:
1. Laser Source
The type of chemical used to dope the laser source impacts the light’s wavelength. Examples include thulium-doped, erbium-doped, and ytterbium-doped fiber lasers. The distinct wavelengths that each of these laser types produces allow them to be employed for different applications.
2. Mode of Operation
Differing laser configurations emit laser beams in different ways. For high peak strengths, laser beams may be "q-switched," "mode-locked," or "gain-switched." They can also be pulsed at a predetermined repetition rate (such as in pulsed lasers). Alternatively, if they were continuous (continuous wave fiber lasers), they might continuously transmit the same quantity of energy.
3. Mode
Mode describes the core size of an optical fiber, which is where the light travels. The two types of fiber laser modes are multi-mode and single-mode. Multi-mode laser core widths often range from 50 to 100 micrometers while single-mode laser core diameters typically come in between 8 and 9 micrometers. Single-mode lasers often provide better beams and more efficient laser light transmission.
4. Laser Power
The term "laser power" refers to the average power of the laser beam, which is given in watts. High-power lasers generate more energy and do so quicker than low-power lasers.
The Parts of a Fiber Laser Machine
A fiber laser machine is a device whose operation depends, at least in part, on a built-in fiber laser system. The core components of a fiber laser cutting machine, for example, include:
- Laser source
- Laser cutting head
- Servo motor
- Cooling device
- Air cutting system
- Laser host (bed, beam, table, and Z-axis system)
- Control system
- Stabilizer
What Are the Types of Fiber Laser Machines?
Table 1 lists some of the top fiber laser marker and engraving machines on the market:
Laser Machine | Power (W) | Material compatibility | Price ($) | Size |
---|---|---|---|---|
Laser Machine Omtech Fiber Laser Marker Engraver | Power (W) 20, 30, 50 | Material compatibility Metals, precious metals, brick and stone, hard plastics | Price ($) $3,099 | Size |
Laser Machine Cloudray AirMarker2 | Power (W) 20 | Material compatibility Metals and some hard plastics | Price ($) $2,100 | Size 110 x 110 mm |
Laser Machine Triumph 3D Fiber Laser Marking Machine | Power (W) 30, 50 | Material compatibility Metals, precious metals, laminates, foils, hard plastics, films, | Price ($) From $4,999 | Size 110 x 100 mm or 210 x 210 mm |
Laser Machine Dihorse Desktop Fiber Laser Marking Machine | Power (W) 20 | Material compatibility Most metals, some nonmetals | Price ($) From $4,769 | Size 110 x 110 mm up to 300 x 300 mm |
Laser Machine Ten-High Portable Fiber Laser Marking Machine | Power (W) 20-50 | Material compatibility Most metals some nonmetals | Price ($) $3,242 | Size — |
Which Industries Use Fiber Lasers?
The following are the top uses for fiber laser cutting equipment:
- Automotive sector
- Manufacturing of home appliances
- Fitness equipment manufacturing
- Manufacturing of lighting
- Manufacturing of decor and metal art
How a Fiber Laser Works
Below are the steps on how a fiber laser works:
1. Creating Light for Lasering
Electricity is converted by laser diodes into photons (light) which are then injected into the fiber optic. The diodes are also referred to as the "pump source" for this reason. Diodes use two oppositely charged semiconductors to produce light. The positively charged electrodes “desire” additional electrons while the negatively charged ones have spare electrons. In order to neutralize the charges, the free electron must jump between electrodes. As it does so, it releases a photon. The number of photons rapidly rises as the semiconductors are subjected to current.
2. Pumping Light Through Fiber-Optic Cables
The fiber core and the cladding are the two fundamental parts that fiber-optic cables utilize to concentrate light in one direction and produce a laser beam. The light travels through the fiber’s core, which is the only section of the cable that contains rare-earth metal and is constructed of silica glass.
3. Amplifying Light in Laser Cavity
When the laser diode light reaches the doped fiber, it interacts with the rare earth atoms, raising the electrons' energy level. This eventually results in a population inversion, which is required for the creation of a typical laser beam.
When a gain medium is in the condition known as "population inversion," more electrons than usual are in an excited state. They will release photons when they drop back to lower energy levels. Some of these electrons can only produce photons with a certain wavelength. When these photons come into contact with other excited electrons, they release comparable photons and drop to their lower energy states. This is the "stimulated emission" part of the acronym LASER (Light Amplification by Stimulated Emission of Radiation).
The incoming light from pump diodes re-excites the electrons as they return to their initial relaxed condition. A constant stream of unprocessed laser light results from the process, eventually reaching a balance between the stimulated and relaxed electrons.
4. Creating Laser Light of Targeted Wavelength
The doping component of the laser cavity affects the fiber’s output wavelength. This is crucial since various applications require different wavelengths. Erbium, thulium, ytterbium, neodymium, and other elements can be used as doping agents. Because each of these elements releases photons at specific energy states, different doping substances create different wavelengths. As a result, all photons produced by a given laser cavity will have the same wavelength. This is why each kind of fiber laser produces that wavelength and nothing else.
5. Shaping Laser Light
Different parts, such as lenses and beam expanders, can be employed to shape the laser beam as desired. Lenses are carefully chosen for different applications according to the advantages that they offer.
For applications where the laser penetrates the material — like laser texturing and engraving — short focal lenses are required. The short focal length concentrates more energy on a smaller region so that it can ablate material.
6. Releasing Laser Light
Finally, after the right lens has been selected, the laser is released to perform the action required. Fiber laser beams can be used to cut, weld, engrave, and clean certain materials (usually metals).
Summary
This article presented information about fiber lasers, explained what they are, and discussed the benefits and uses of a fiber laser.
Xometry provides a wide range of manufacturing capabilities, including sheet cutting and other services for all of your prototyping and production needs. Visit our website to learn more or to request a instant quote.
Disclaimer
The content appearing on this webpage is for informational purposes only. Xometry makes no representation or warranty of any kind, be it expressed or implied, as to the accuracy, completeness, or validity of the information. Any performance parameters, geometric tolerances, specific design features, quality and types of materials, or processes should not be inferred to represent what will be delivered by third-party suppliers or manufacturers through Xometry’s network. Buyers seeking quotes for parts are responsible for defining the specific requirements for those parts. Please refer to our terms and conditions for more information.
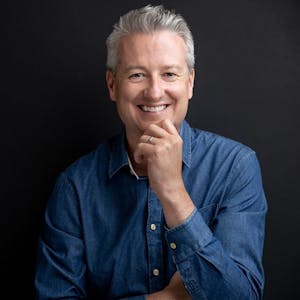